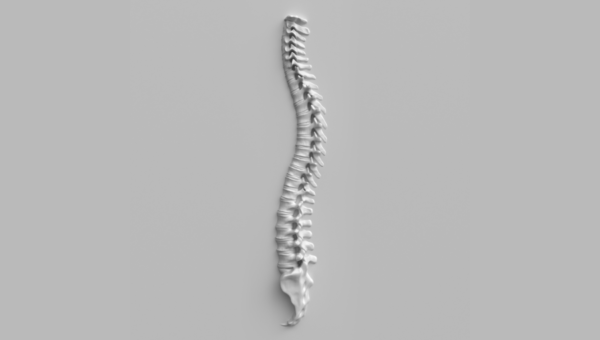
MRM Insights: A Mouse Model for Studying Neurogenic Heterotopic Ossification in Spinal Cord Injury
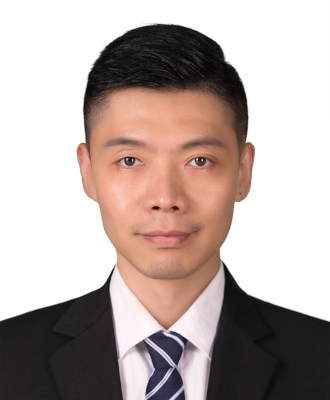
Chan Gao
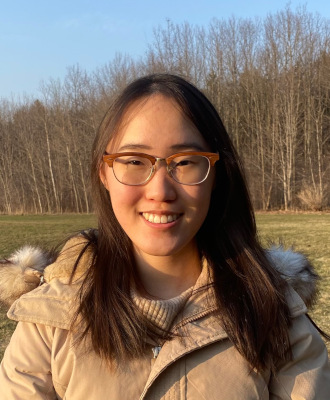
Ivy Sun
Every month, in the MRM Insights, a member of the MRM Network offers a unique perspective on the topics of stem cells and regenerative medicine. In this edition, Dr. Chan Gao, who serves as an Assistant Professor in the Departments of Medicine and Surgery, and Junior Scientist at the RI-MUHC, collaborates with Ivy Sun, an undergraduate research intern in his lab. Together, they present a mouse model for elucidating the mechanisms underlying spinal cord injury-associated neurogenic heterotopic ossification.
A Mouse Model for Elucidating the Mechanisms Underlying Spinal Cord Injury-associated Neurogenic Heterotopic Ossification
Heterotopic ossification (HO) is ectopic bone formation in soft tissues like muscle and tendon that causes pain, joint stiffness, and neurovascular compromise. Examples of injuries conducive to HO include those sustained in traffic accidents and falls, surgical replacement or reconstruction of joints, and repetitive overuse of muscle and tendon by elite athletes. HO is conceptualized as a form of aberrant tissue regeneration and repair characterized by prolonged inflammation. Available therapeutics for HO, including non-steroidal anti-inflammatory drugs (NSAIDs), bisphosphonate, and radiation therapy, are largely ineffective and raise safety concerns for long-term use.

Mouse model of SCI-associated NHO. Sensory neurons of dorsal root ganglion (DRG) from SCI+MTI mice exhibit distinct morphological characteristics when compared to those extracted from naïve Control mice. DRG neurons from Control mice (A) show normal neuronal bodies (blue arrows) and axonal connections (green arrows). DRG neurons from SCI+MTI mice (B) are characterized by abnormal cell bodies (orange arrows) and disrupted axonal connections (red arrows). Undecalcified bone stained with Von Kossa (upper) shows NHO formed in the damaged quadriceps with concomitant loss of orthotopic bone in SCI + MTI mice at postoperative 3 weeks (D) compared with naïve Control (C). Safranin O-stained adjacent sections (lower) show abnormal cartilage in the SCI+MTI knees. High magnification of ectopic bone (yellow square) in SCI+MTI knees shows organized columns of chondrocytes reminiscent of developing growth plate.
HO resulting from injuries to the central nervous system (CNS), i.e., brain and/or spinal cord, is called neurogenic HO (NHO). NHO was first recognized as a frequent and serious complication of traumatic spinal cord injury (SCI) in paraplegic veterans of World War I1. It is now diagnosed in more than 20% of individuals living with the consequences of severe SCI2 in which affects the muscles and tendons around big joints. Like orthotopic bone, ectopic bone is proposed to develop from progenitor cell condensation and osteogenic differentiation under the influence of local cytokines and growth factors11,12. Previous work using in vivo models has implicated endothelial cells, muscle fibro/adipogenic progenitors (FAPs), pericytes, and tenocyte precursors in HO3-7. Others suggest circulating osteogenic precursors (COP) in peripheral blood home to sites of bone remodeling and skeletal muscle injury to form ectopic bone8,9. This hypothesis is supported by clinical data documenting HO in specimens harvested from patients with predisposing conditions including CNS injuries10.
In the absence of a clinically relevant model to investigate pathogenetic mechanisms underlying SCI-associated NHO, we recently developed a mouse that mimics the clinical presentation of severe traumatic SCI with concomitant myotendinous injuries (SCI+MTI). Young adult mice undergo complete SCI by spinal cord transection at T9-10 followed by quadriceps muscle crush and tenotomy. Phenotypic characterization using histological analyses reveal ectopic bone and cartilage in the damaged quadriceps with concomitant loss of orthotopic bone in SCI+MTI mice euthanized at postoperative 3 weeks (Figure). Initial analysis of sensory neurons isolated from lumbar dorsal root ganglion (DRG) removed from below the SCI at postoperative 3 days reveal altered morphology. While this work associates sensory neurogenesis with osteogenesis in the context of SCI-associated NHO, the molecular pathways remain undefined. Further insight into those pathogenic mechanisms in our model will be obtained from unbiased proteomic profiling.
Proteomic analyses have been conducted on tissue specimens from patients with ossification of the posterior longitudinal ligament, thoracic ossification of ligamentum flavum and with traumatic HO. Statistically significant differences were demonstrated in pathways involved in inflammation and immunity, lipid metabolism, reactive oxygen species metabolism, angiogenesis, osteoblast differentiation of mesenchymal stem cells, and extracellular matrix mineralization17-19. Another pre-clinical study revealed dysregulation of TGFβ, MAPK, coagulation, and oxidative stress pathways in a rat model of skeletal muscle injury20. We will undertake a comprehensive proteomic analysis of DRG sensory neurons and quadriceps myotendinous tissue obtained from SCI+MTI mice. The approach will identify potential molecular targets for the development of novel diagnostic, prognostic and therapeutic applications.
References
1. Eisenstein N, Stapley S, Grover L. Post-traumatic heterotopic ossification: an old problem in need of a new solution. J Orthop Res. 2018;April 2018:1061-1068.
2. Sullivan MP, Torres SJ, Mehta S, Ahn J. Heterotopic ossification after central nervous system trauma: A current review. Bone Joint Res. Mar 2013;2(3):51-7. doi:10.1302/2046-3758.23.2000152
3. Wosczyna M, Biswas A, Cogswell C, Goldhamer D. Multipotent progenitors resident in the skeletal muscle interstitium exhibit robust BMP-dependent osteogenic activity and mediate heterotopic ossification. J Bone Miner Res. 2012;27(5):1004-1007.
4. Lees-Shepard J, Goldhamer D. Stem cells and heterotopic ossification: lessons from animal models. Bone. 2018;109:178-186.
5. Agarwal S, Loder S, Cholok D, et al. Scleraxis-lineage cells contribute to ectopic bone formation in muscle and tendon. Stem Cells. 2017;35(3):705-710.
6. Liu H, Jiang R. MKX-deficient mice exhibit hedgehog signalling-dependent ectopic ossification in the achilles tendons. J Bone Miner Res. 2019;34(3):557-569.
7. Tirone M, Giovenzana A, Vallone A, et al. Severe Heterotopic Ossification in the Skeletal Muscle and Endothelial Cells Recruitment to Chondrogenesis Are Enhanced by Monocyte/Macrophage Depletion. Frontiers in immunology. 2019;10:1640. doi:10.3389/fimmu.2019.01640
8. Otsutu S, Tamai K, Yamazaki T, Yoshikawa H, Kaneda Y. Bone marrow-derived osteoblast progenitor cells in circulating blood contribute to ectopic bone formation in mice. Biochem Biophys Res Comm. 2007;354:453-458.
9. Jaerve A, Bosse F, Muller H. SDF-1/CXCL12: its role in spinal cord injury. Int J Biochem & Cell Biol. 2012;44:452-456.
10. Egan K, Duque G, Keenan M, Pignolo R. Circulating osteogenic precursor cells in non-hereditary heterotopic ossification. Bone. 2018;109:61-64.
11. Erlebacher A, Filvaroff EH, Gitelman SE, Derynck R. Toward a molecular understanding of skeletal development. Cell. 1995;80:371-378.
12. Shore E, Kaplan F. Inherited human diseases of heterotopic bone formation. Nat Rev Rheumatol. 2010;6(9):518-527.
13. Tomlinson R, Christiansen B, Giannone A, Genetos D. The role of nerves in skeletal development adaptation and aging. Frontiers Endocrinology. 2020;11(Article 646)
14. Brazill J, Beeve A, Craft C, Ivanusic J, Scheller E. Nerves in bone: evolving concepts in pain and anabolism. J Bone Miner Res. 2019;34:1393-1406.
15. Sisask G, Silfverswärd CJ, Bjurholm A, Nilsson O. Ontogeny of sensory and autonomic nerves in the developing mouse skeleton. Auton Neurosci. Oct 2013;177(2):237-43. doi:10.1016/j.autneu.2013.05.005
16. Lee S, Hwang C, Marini S, et al. NGF-TrkA signaling dictates neural ingrowth and aberrant osteochondral differentiation after soft tissue trauma. Nature communications. 2021/08/16 2021;12(1):4939. doi:10.1038/s41467-021-25143-z
17. Oh YM, Lee WJ, Kim MG, Ma TZ, Kwak YG, Eun JP. Comparative proteomic tissue analysis in patients with ossification of the posterior longitudinal ligament. World Neurosurg. Jul-Aug 2014;82(1-2):e353-9. doi:10.1016/j.wneu.2013.03.033
18. Crowgey EL, Wyffels JT, Osborn PM, Wood TT, Edsberg LE. A Systems Biology Approach for Studying Heterotopic Ossification: Proteomic Analysis of Clinical Serum and Tissue Samples. Genomics Proteomics Bioinformatics. Jun 2018;16(3):212-220. doi:10.1016/j.gpb.2018.04.006
19. Wang B, Chen Z, Meng X, Li M, Yang X, Zhang C. iTRAQ quantitative proteomic study in patients with thoracic ossification of the ligamentum flavum. Biochem Biophys Res Commun. Jun 10 2017;487(4):834-839. doi:10.1016/j.bbrc.2017.04.136
20. Hoover ME, Martin EC, Llamas CB, et al. Proteomic characterization of a trauma-based rat model of heterotopic ossification identifies interactive signaling networks as potential therapeutic targets. Journal of proteomics. Aug 30 2020;226:103907. doi:10.1016/j.jprot.2020.103907