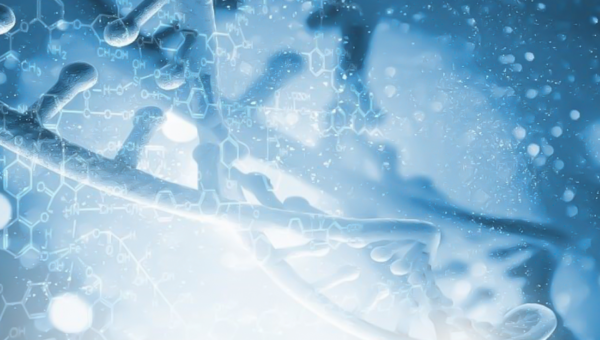
Des chercheurs de McGill perfectionnent des outils d’édition du génome
La version française sera disponible bientôt
The complex task of genome editing is continuously evolving thanks in part to efforts by a team of researchers in the Department of Pharmacology and Therapeutics at McGill University’s Faculty of Medicine and Health Sciences, led by Professor Moshe Szyf and PhD student Daniel M. Sapozhnikov.
This story was written by Timothy Gouldson, and originally published on McGill Health e-News.
Front and centre of gene-editing technology is Clustered Regularly Interspaced Short Palindromic Repeats/CRISPR-associated protein 9, better known as CRISPR/Cas9, a powerful tool whose origin lies in the natural adaptive immunity of bacteria. CRISPR/Cas9 edits genes by precisely cutting DNA and then letting natural DNA repair processes take over, creating transformative therapies.
At the moment however, a concern is long-term safety. CRISPR/Cas9 tolerates sequence mismatches and edits off-target sites at a high frequency, thereby posing the significant clinical risk of producing adverse effects by unintended mutagenesis. In other words, CRISPR/Cas9 damages DNA in places that you don’t want to damage.
“The way CRISPR/Cas9 works is you send it to any place in the genome based on approximately 20-base pairs of identity. Any place in the genome that has that 20-base pair sequence will get targeted by CRISPR for gene editing purposes,” explains Sapozhnikov, the first author on a recent publication in Scientific Reports. “The problem with that is those 20 base pairs tolerate a bit of difference. If there are places that are similar in sequence to those 20 base pairs, but a few base pairs off, the CRISPR/Cas9 complex might go there, even though that’s not where you intended it to go, and it’ll cut the DNA there and potentially cause some mutations.”
To mitigate this deviation, the team developed a method called PROTECTOR, (PRevention of Off-Target Effects of CRISPR/Cas by Thwarting ORthologs). It works by using a different form of CRISPR/Cas9 – called dead Cas9 or dCas9 – which can be targeted to specific places in the DNA but has no ability to change the DNA sequence. Here, dCas9 is specifically targeted to known off-target sites so that it physically obstructs the active form of CRISPR/Cas9 from being able to access the off-target site, leaving only the true target available for modification.
“Our strategy uses CRISPR, but from a different species, so it doesn’t interfere with the active CRISPR you’re using. And that CRISPR that we’re using from the other species is catalytically dead. So, it will go wherever those 20 base pairs you send it to are, but it won’t cut the DNA there, and by doing that, by placing it so it sits there at these off-target sites that are similar to the site you’re really targeting, it will prevent the active form of CRISPR from being able to access the DNA because you have this inactive one taking up that space,” notes Sapozhnikov.
One example is the CCR5 gene, which encodes a receptor that is critical for HIV to be able to infect human cells. Modifying the receptor by introducing a mutation in the CCR5 gene can produce cells that are resistant to infection by HIV, but the sequence of CCR5 is similar to another important receptor – CCR2 – and unintended mutations that are often produced by CRISPR/Cas9 are at CCR2, potentially leading to unwanted health effects. “Here, we show that PROTECTOR dramatically reduces the frequency of off-target mutations at CCR2 while actually increasing the frequency of successful mutation of CCR5,” Sapozhnikov says.
The PROTECTOR strategy can be combined with other new off-target reduction methods – such as more accurate, but imperfect, artificially improved CRISPR/Cas9 proteins that are less prone to modify off-targets – to further improve the landscape of off-target effects of these more accurate systems.
But does that mean the risk of off-target mutations is eliminated? Not exactly. There is still work to be done. To get to that point Sapozhnikov says, requires further research on the part of the scientific community. “There’s still a lot of people who are trying to make better CRISPR enzymes, artificial enzymes that behave like CRISPR but are higher fidelity. They don’t go to these off-targets as much. Other things to optimize would be ratios: how much PROTECTOR are you adding, how much CRISPR are you adding? With any drug and its delivery system, there’s a maximum dose that can be delivered and tolerated. Obviously, if you add more PROTECTOR, you’re biasing towards protecting those off-target sites better versus the catalytically active one. But then the question becomes will there be enough catalytically active CRISPR/Cas9 to still be efficient in doing what you want it to do in the first place? The two biggest routes to bring it down, to eliminate off-target mutation if possible, would be to optimize the ratios of the components and then invoke the PROTECTOR strategy in tandem with other known strategies to reduce off-targets.”
CRISPR still holds promise in combatting several disorders, such as HIV, sickle cell anemia, and hereditary blindness, and it already has. “It comes down to, is the disease caused by a genetic predisposition, and can CRISPR correct it. Separate from PROTECTOR, we are already using a dead Cas9 for other purposes. So instead of protecting it from CRISPR like PROTECTOR does, we were protecting DNA from getting chemically modified. It’s called DNA methylation. A chemical called a methyl group gets added to the DNA and that changes the way that genes are turned on and off,” Sapozhnikov explains.
DNA methylation involves the enzymatic addition of a methyl group primarily to cytosine residues in DNA. In another recent paper in Nature Communications, Sapozhnikov explains how the technique works. “The cells of a human body, despite having the same DNA, are specialized – like those of the heart or of the lungs – and that’s achieved by selectively turning on and off the genes needed for that cell’s specific function. Genes with a lot of that methyl group near their start sites tend to be off, so what dCas9 can do is sit near those start sites and prevent the cell’s natural machinery from adding those methyl groups. When that’s done at the right time, dCas9 can be removed, and now you have a gene that is not silenced by methylation. This technique is useful now to study how DNA methylation works to turn genes off, but it could be useful therapeutically, to turn back on genes that were wrongfully silenced during the development of a disease.”
To learn more, see The PROTECTOR strategy employs dCas orthologs to sterically shield off-target sites from CRISPR/Cas activity, published in Scientific Reports in February 2023.