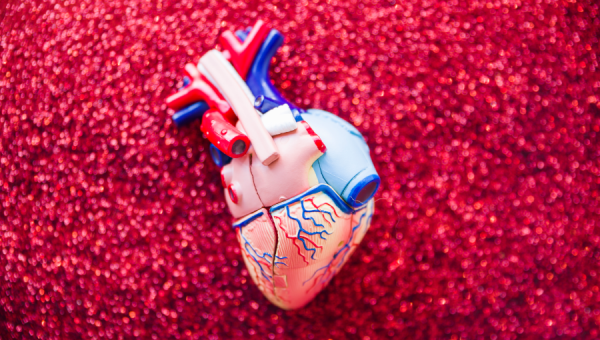
MRM Insights: Modelling cardiomyopathies and rare diseases using patient-derived iPSCs

Professor Terry Hébert
Every month, in MRM Insights, a member of the MRM Network is writing about stem cells and regenerative medicine from a different perspective. This month, Dr. Terry Hébert, Professor in the Department of Pharmacology and Therapeutics, Assistant Dean of Biomedical Science Education, member of the MRM Executive Committee and Chair of the MRM Education Committe, talks about modelling cardiomyocytes and rare diseases using patient-derived iPSCs.
Modelling cardiomyopathies and rare diseases using patient-derived iPSCs – stem cells leading to precision medicine
Precision medicine represents the future of personalized healthcare and health innovation in Canada and beyond. To make headway in this area, we need to combine basic, clinical and translational research, data and investigators to create unique platforms to understand, treat and hopefully cure diseases. This is true for diseases that affects millions of people and for diseases that affect hundreds of people.
Common diseases: different faces
Cardiovascular disease remains the leading cause of death in the western world- to give you a sense of the problem 1 in 4 women will get heart disease while 1 in 40 will be stricken with breast cancer. Of particular clinical importance are diseases known as cardiomyopathies, a disease of the heart muscle that reduces the heart’s ability to pump oxygenated blood to the body. This can lead to heart failure, a cardiovascular condition with the fastest rising incidence and a 5-year survival rate of only 50%. Importantly, various disease states causing cardiac remodeling and hypertrophy can be modeled using patient-derived, iPSC-based 2D and 3D culture systems. Blood cells can be expanded and turned into iPSCs, which can also be biobanked, facilitating their use for modelling the effects of specific genes on the development of heart disease and to testing whether particular therapeutic approaches might work better in specific patients – an approach that has been validated in studies of cardiomyopathies. Based on patient-derived iPSCs, we have recently developed a program called the Courtois Cardiomyopathy Initiative we hope will revolutionize preclinical modelling for drug development using patient-derived inducible pluripotent stem cells (iPSCs) and organoids which are cell-derived 3D organotypic models for the study of disease processes and therapeutic responses.
With colleagues at the McGill University Health Centre (MUHC), Dr. Nadia Giannetti and Dr. Renzo Cecere, we have now established a pipeline allowing us to obtain blood samples from large cohorts of patients with diagnosed cardiomyopathies. This will allow us to conduct large scale iPSC-based modelling studies, providing an opportunity to personalize treatments for a range of clinically important cardiovascular diseases. Over the past few years, we have helped conceive, design, produce and validate a large number of bioluminescence- and fluorescence resonance energy transfer (BRET and FRET)-based biosensors to track receptor signalling by measuring activation of heterotrimeric G proteins, downstream effectors, production of second messengers and the recruitment of GPCR-interacting proteins. Biosensor outputs depend critically on cellular and subcellular contexts- and to be honest, a lot of drug discovery starts in irrelevant heterologous cell culture systems that do not reflect either the disease or the tissues affected. Here, our aim is use biosensors to examine GPCR signalling in a cell context-specific way in different cells in the myocardium and vasculature. Our iPSC-based platforms give us the means to study such signalling events in multiple cell types relevant to the cardiovascular system as well as other cell types.
We hope that the ability to model disease progression in individual cell types, co-cultured primary and iPSC-derived cells and organoid models will provide an innovative and unique venue in which to use our established biosensor-based and phenotypic approaches to drug discovery. To date, we have extensively phenotyped iPSC lines and generated cardiomyocytes and vascular smooth muscle cells from them. We hope that our program will feed disruptive development of personalized treatment to improve patient outcomes. This work brings together clinician scientists and molecular pharmacologists and ultimately tissue engineers like Dr. Corinne Hoesli in an ambitious framework to tackle personalized aspects of cardiovascular disease.
The recent creation of the Courtois Cardiovascular Signature Program provides a local framework for all aspects of this work. To optimize translational outputs from such research into different cardiomyopathies, we need a richer and more complete understanding of patients in the real world. Our goal here is to develop better preclinical models linking clinical samples with state-of-the art drug screening technologies using patient-derived inducible pluripotent stem cells (iPSCs) and ultimately 3D versions of these cells known as organoids. These will feed the disruptive development of personalized treatment with better outcomes for patients, through improved understanding of individualized disease mechanisms and therapeutic responses, new molecular diagnostics and targeted therapies. To truly personalize medicine, we must be able to model disease on a patient-specific basis. To reach this point we need 1) patient-derived blood samples, 2) iPSC generation and validation, 3) phenotypic validation of iPSC-derived cells such as cardiomyocytes (click the link), fibroblasts, endothelial cells and vascular smooth muscle cells from patients with cardiovascular disease and 4) biosensor platforms to capture unique patient- and disease-specific signalling events in iPSCs and corresponding derived differentiated cells in 2D and 3D cultures. This particular combination of biosensor approaches to drug discovery and disease modelling in iPSCs is unique to McGill University.
Our initiative is part of a larger endeavour begun by Dr. Gianneti called The Courtois Cardiovascular Signature Biorepository. This is a collection of biological specimens and clinical data from participants with or at risk of acquired heart disease, their family members and healthy participants as controls. This project will generate critical new scientific data leading to improved treatments for cardiovascular disease. Patients over 18 years of age who present to the MUHC with newly diagnosed cardiomyopathy or who are already followed in the heart failure clinic of the MUHC with a diagnosis of cardiomyopathy are eligible. There are presently 800 active patients followed at the heart failure clinic. Our program will develop personalized medicine approaches for patients with cardiomyopathies, both genetic and idiopathic. For our part, we have been generously supported by the Courtois Foundation to fund the initial generation of iPSC lines from 100 patients from this pool, which will enable us to establish proof-of-principle for our pipeline. Increased throughput of stem cell generation and differentiation will be developed further support, including an expanded ability to collect and biobank patient-derived samples.
Rare disease: rare opportunity
Imagine life as a parent whose child is afflicted by a rare disease. As of 15 years ago, doctors could only treat symptoms, the pharmaceutical industry was not interested and parents and child faced an uncertain future together. Now with the advent of whole genome sequencing and stem cell-based modelling of disease, patients and their advocates can focus on understanding how these diseases work, connect with other families with the same disease and invest their time and energy in more productive advocacy aimed at better treatments and even cures. Beyond this, we owe patients with rare disease a tremendous debt- the mutations in individual genes that affect these individuals provide a powerful lens to understand how these genes work under normal conditions- which can often translate into therapeutic approaches to diseases that are much more common. One such example is the story of the blockbuster drugs called statins used to treat patients with high cholesterol. The discovery of these drugs was based on studies of a handful of patients with a rare familial hypercholesterolemia by Michael Brown and Joseph Goldstein. Learning about how cholesterol transport and synthesis worked using cells from these patients transformed our understanding of how to treat millions of patients with high cholesterol and its consequences for heart disease and stroke. It also resulted in a Nobel Prize for Brown and Goldstein in 1985 and led to the development of statins, drugs which affect the balance of cholesterol mobilization and help remove blood-borne cholesterol from the circulation. They did all this work before the advent of the human genome project- which is even more amazing. Imagine now how an understanding of other rare diseases might translate into similar development of new medicines for other diseases such as cancer, heart disease and immune disorders. We have reached a critical point in making this possible.
The power of iPSC-based approaches is also strongly manifested when considering patients suffering from rare diseases. A number of recent studies have shown that similar mutations that occur in the germline (in more than 80 patients to date) lead to developmental delay, autism-like symptoms, seizures and hypotonia. Such mutations are found in several important effector binding surfaces of Gβ subunits but these have not been systematically tested. Germline loss of Gβ1 is embryonic lethal. We will focus on germline point mutations in the Gβ1 subunit, encoded by the gene GNB1, studying the effects of these mutations in vitro, with a view toward understanding their impact on signalling in different cell types such as neurons, where their impact is felt. Our objectives are to map functional regions, and connect functional outcomes to clinical phenotypes.
Our work addresses G protein signalling and the impact of GNB1 mutations in iPSC-based models relevant to this rare neurodevelopmental disease. Although we will take an approach that examines previously known GPCR/G protein signalling events, such as the regulation of ion channels in the heart and brain, an important focus will be on events that occur in the nucleus of cells, where Gβγ signalling might control how genes are turned on or off, efforts we have pioneered and are uniquely poised to study. Understanding their impact, will guide us to personalized therapeutic strategies that might help GNB1 patients both individually and collectively. We will be able to use our models to personalize treatments for each patient but we will also create an amazing resource to understand the broader roles of genes mutated in these patients. We have already developed lines obtained from patients with germline mutations in GNB1 which cause this neurodevelopmental phenotype along with control lines from family members.
My sense is that the future of personalized and precision medicine is happening right before our eyes now. At the end of the day, for me personally, it has been a deeply affecting process. As a basic scientist, we often dream of discovering the secrets of nature, whatever that means. Interacting with the patients, families and healthcare providers for cardiomyopathy and for GNB1 has transformed how I see my research unfolding. I want to help. The use of iPSCs derived from patients has provided my lab with the opportunity to do this. I am deeply grateful for the funding, yes- but also deeply grateful for the new meaning my work has for me and my students. Like I said, we want to help.
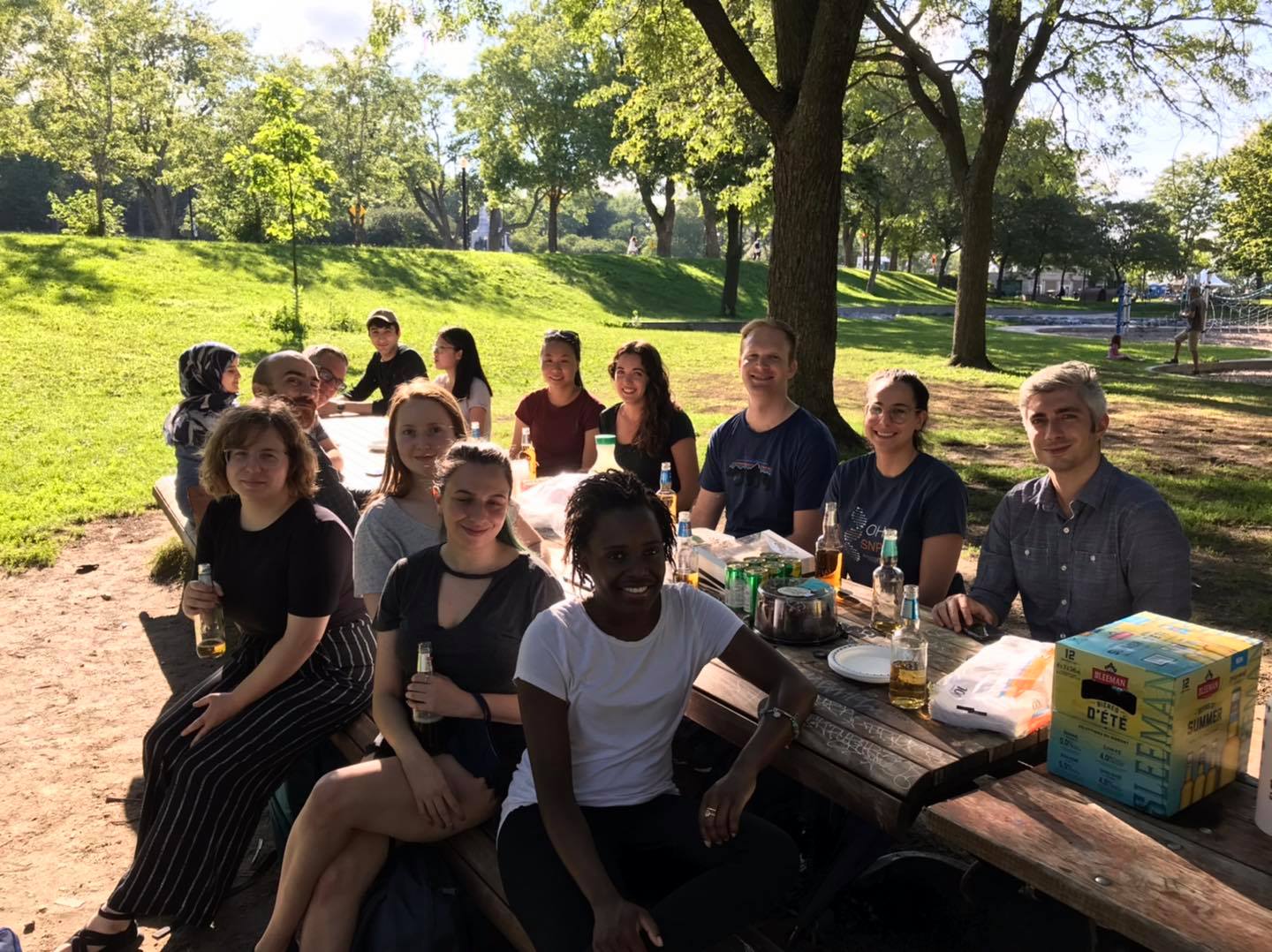
The Hébert Lab
Heart photo created by freepik – www.freepik.com