
MRM Insights: Studying muscle stem cells within the context of their niche environment during development and diseases
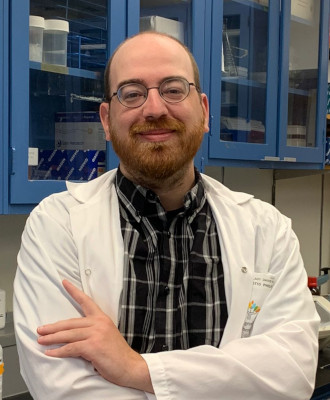
Darren Blackburn

Dr. Vahab Soleimani
Every month, in MRM Insights, a member of the MRM Network is writing about stem cells and regenerative medicine from a different perspective. This month, Dr. Vahab Soleimani, Associate Professor in the Department of Human Genetics at McGill University and Investigator at the Lady Davis Institute, and Darren Blackburn, Ph.D. candidate in his lab, are discussing how to study muscle stem cells within the context of their niche environment.
Studying muscle stem cells within the context of their niche environment during development and diseases
Skeletal muscle is the largest tissue in the human body and has evolved for the generation of force1. Within this tissue resides a rare adult stem cell population known as satellite cells or muscle stem cells (MuSCs). These cells are absolutely required for the regeneration and lifelong maintenance of skeletal muscle2,3. Under normal conditions, MuSCs will remain in a quiescent state4. However, when the muscle is injured, they will quickly respond by activating, transiently proliferating, and differentiating, while some will return to quiescence replenishing the stem cell pool. When the signal to activate arrives, the MuSCs will initiate the myogenic program through the tightly regulated temporal expression of the four myogenic factors: Myf5, MyoD, Myogenin and Myf65. Myf5 and MyoD are the first of the factors to be expressed, promoting the proliferation of MuSCs and transforming them into cycling myoblasts6,7. Afterwards, MyoD and Myogenin will be expressed in conjunction, pushing the myoblasts into terminal differentiation as myocytes, as well as allowing for the fusion of these cells to form myotubes, or to fuse with preexisting myofibers8. In the mature myofibers, Myf6 will be the final myogenic factor expressed9.
Adult stem cells are known to exist within a niche environment that maintains their population and will regulate their function10. The concept of a niche environment for adult stem cells was first hypothesized in 197811. A niche is more than a physical location, but also a nexus point where extrinsic signals can gather and interact with the stem cells and should the stem cell be removed from this niche, the result is differentiation or cell death. MuSCs are no exception and exist within a clearly defined physical niche, residing in between the basal lamina and sarcolemma of myofibers12. In fact, it is due to this unique location that first allowed MuSCs to be hypothesized as being adult stem cells, and are the only adult stem cells to have been initially identified due to their anatomical location, instead of their biological function.
The MuSC niche is complex and composed of a large number of different cell types12. In addition, the niche is a dynamic environment and changes during the course of diseases and during normal aging. A major component of the MuSC niche is the myofiber itself. In addition to being the cell that the MuSC is nestled on, the myofiber also plays a signaling role, producing a number of growth factors and myokines that directly interact with the MuSC. An example of this is the growth factor EGF, which is produced by the myofiber and is under the transcriptional control of Myf69. EGF affects MuSCs by, among other things, promoting asymmetrical division, allowing for the MuSC pool to be maintained13. Further, the myofiber also produces ligands involved in crucial pathways, including Notch and WNT ligands14.
Other crucial components of the niche include non-myogenic cells such as Fibro/Adipogenic Progenitors (FAPs), macrophages, endothelial cells and fibroblasts among others. It is known that FAPs produce many components of the extracellular matrix (ECM) and that the stiffness of the ECM is one of the factors that influence MuSC behaviour. In addition, FAPs support MuSCs during muscle regeneration, secreting a number of factors that will either directly affect the MuSCs, for example WISP-1 which promotes the proliferation of MuSCs by activating the AKT pathway, or to influence other cell types, such as the secretion of IL-33 which assists in T-cell recruitment, which are needed for proper muscle regeneration15,16. Macrophages, beyond their well-established role in clearing debris and breaking down damaged tissue, also communicate directly with MuSCs. Macrophages are classically divided into two subgroups: M1, which are viewed as pro-inflammatory, and M2 that are anti-inflammatory17. During regeneration, macrophages are primarily M1, and these cells secrete cytokines that promote the proliferation of MuSCs, including IGF-118. When the transition to M2 macrophages occurs, the secreted cytokines also transition from promoting proliferation to ones that encourage differentiation of the MuSCs, such as GDF319. Beyond their effect on MuSCs, macrophages and FAPs also interact with each other to maintain the MuSC niche. During the early stages of regeneration, the M1 macrophages induce apoptosis in the FAPs by secreting TNF, this action prevents the accumulation of FAPs and fibrosis20. However, during the later stages of regeneration the M2 macrophages produce TGF-Beta, which stops the clearance of the FAPs and allows the tissue to return to a state of homeostasis20.
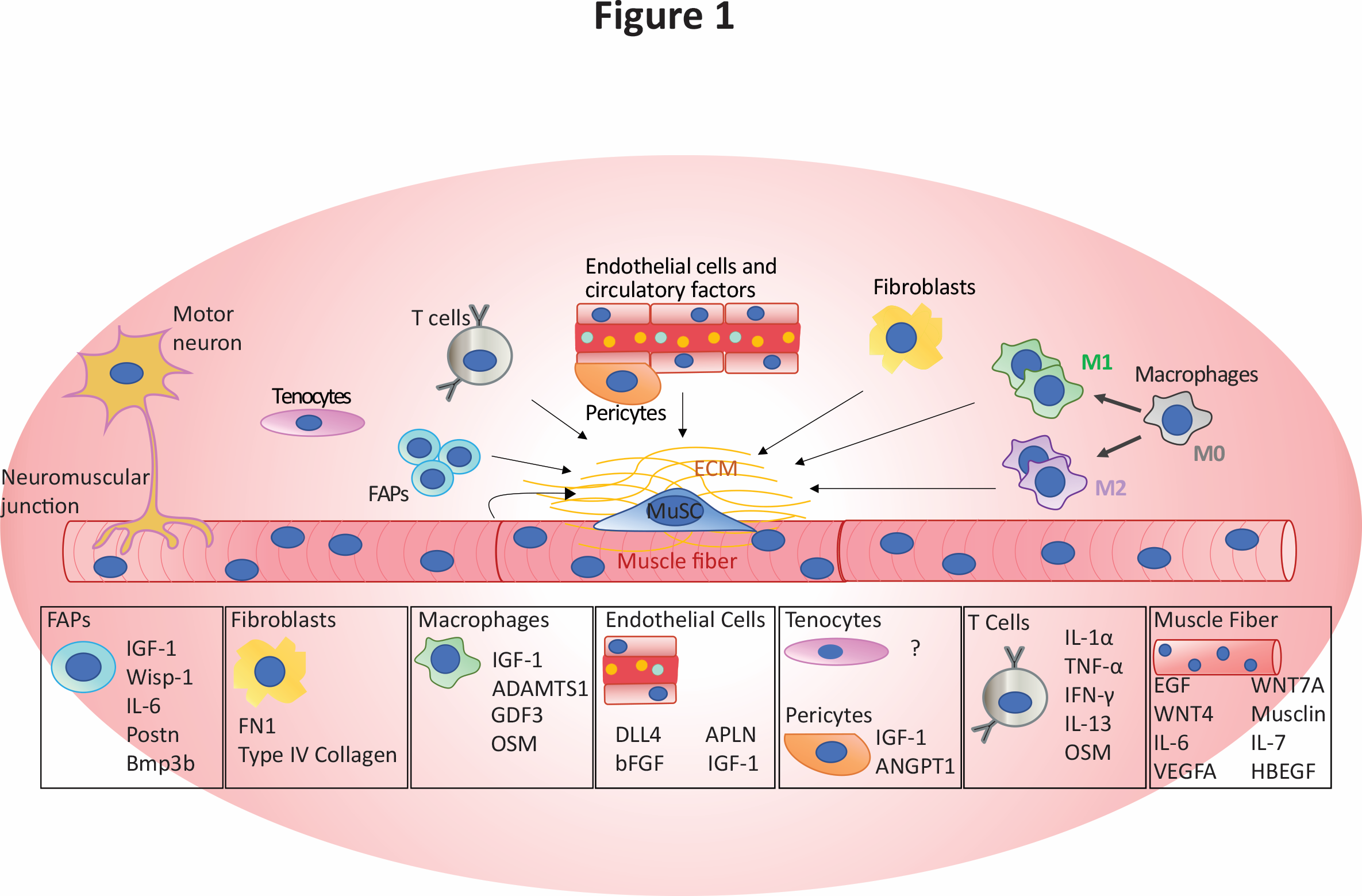
Figure 1: Components of the MuSC niche and secreted factors known to influence the MuSCs and other niche elements, retrieved from Blackburn et al. 2021, Critical Reviews in Biochemistry and Molecular Biology 202121.
Although MuSCs have a remarkable capacity to regenerate damaged tissue, aging has a detrimental effect on both MuSCs and their niche environment22. While there are many age-related cell-intrinsic changes in MuSCs that contribute to the loss of their regenerative potential, there are also major contributing factors arising from the MuSC niche that have an effect on the stem cells during the course of disease and aging. It is well established that when humans age the skeletal muscle will exhibit an increase in fibrosis and fat infiltration23,24. Beyond that, the members of the niche will undergo changes in numbers, function, and subtype, all of which can have negative effects on MuSC function. For example, myofibers in aged mice produce higher levels of FGF2, which reduces the ability of MuSCs to remain quiescent and could lead to a loss of the stem cell pool25.
Since the niche plays a critical role in regulating MuSC function, it is crucial to study them within the context of their niche environment. Removal of MuSCs from their niche environment and their ex vivo expansion leads to significant reduction in their stemness, thereby providing researchers with data that may not be reflective of their normal behaviour. While the gold standard for research would be to conduct studies in vivo, one of the great challenges with MuSCs is their rarity, and traditional sequencing methods were not up to the task of fully capturing their transcriptome and epigenome. In addition to the rarity of MuSCs themselves, the skeletal muscle niche is also very complex and heterogenous. Bulk RNA-Seq will be able to capture the average expression across tissue, but will be unable to ascribe genes to specific cell types, making it challenging to design experiments to capture cell-specific transcriptome and epigenome.
Recent advances in Next Generation Sequencing technologies, including single cell sequencing, now permits rare cell types to be analyzed and characterized both at the bulk as well as at the single cell level. These advancements were made possible by the development of Switching Mechanism at 5′ end of RNA Template (SMART) and Tn5-based tagmentation technologies and allows one to bypass the limitation on low cell number26.
It is expected that the application of these powerful tools will allow researchers to greatly improve the understanding of how adult stem cells behave within the context of the niche and how this interaction is altered in ageing and disease contexts. In recent years, these technologies have already been applied to assess how the niche and MuSCs interact during acute injury, and also how the niche can affect MuSC function in ageing. Recently, a paper utilized single cell RNA-Seq to characterize the muscle niche during the stages of acute injury, revealing an incredibly dynamic niche that quickly changes in response to injury and also rapidly returns to homeostasis after regeneration27. Further, another study using scRNA-Seq in young and aged mice revealed that in the aged muscle there is an increase in the number of FAPs, which may contribute to increased ECM deposition and stiffness, an increase in stiffness can negatively impact MuSC function28,29. During aging there is also a change in the subtype of macrophages, having a shift from anti-inflammatory macrophages towards pro-inflammatory28. The advent of SMART technology also allows for the sequencing of minute samples, which was previously unfeasible, such as whole transcriptome analysis of a single myofiber14,26.
Although single cell RNA sequencing is a powerful tool to determine the heterogeneity in cell populations within a tissue, it does not, however, by itself capture the spatial distribution of specific cells or potential cell to cell communication30. Recent advances in spatial transcriptomics such as high-plex RNA imaging or spatial barcoding can be used to localize specific cells within a defined anatomical location in a tissue30. Combining the latter tools with single cell technologies and high throughput computational methods is expected to provide detailed insights into how adult stem cells communicate with their niche environment during development and diseases. This new knowledge will serve as a foundation to develop novel therapies for treatment of muscle wasting diseases such as age-related sarcopenia and neuromuscular disorders.
References
1 Janssen, I., Heymsfield, S. B., Wang, Z. M. & Ross, R. Skeletal muscle mass and distribution in 468 men and women aged 18-88 yr. J Appl Physiol (1985) 89, 81-88, doi:10.1152/jappl.2000.89.1.81 (2000).
2 Le Grand, F. et al. Six1 regulates stem cell repair potential and self-renewal during skeletal muscle regeneration. J Cell Biol 198, 815-832, doi:10.1083/jcb.201201050 (2012).
3 Wang, Y. X. & Rudnicki, M. A. Satellite cells, the engines of muscle repair. Nat Rev Mol Cell Biol 13, 127-133, doi:10.1038/nrm3265 (2011).
4 Schultz, E., Gibson, M. C. & Champion, T. Satellite cells are mitotically quiescent in mature mouse muscle: an EM and radioautographic study. J Exp Zool 206, 451-456, doi:10.1002/jez.1402060314 (1978).
5 Braun, T., Bober, E., Winter, B., Rosenthal, N. & Arnold, H. H. Myf-6, a new member of the human gene family of myogenic determination factors: evidence for a gene cluster on chromosome 12. EMBO J 9, 821-831 (1990).
6 Davis, R. L., Weintraub, H. & Lassar, A. B. Expression of a single transfected cDNA converts fibroblasts to myoblasts. Cell 51, 987-1000, doi:10.1016/0092-8674(87)90585-x (1987).
7 Weintraub, H. et al. Activation of muscle-specific genes in pigment, nerve, fat, liver, and fibroblast cell lines by forced expression of MyoD. Proc Natl Acad Sci U S A 86, 5434-5438, doi:10.1073/pnas.86.14.5434 (1989).
8 Hasty, P. et al. Muscle deficiency and neonatal death in mice with a targeted mutation in the myogenin gene. Nature 364, 501-506, doi:10.1038/364501a0 (1993).
9 Lazure, F. et al. Myf6/MRF4 is a myogenic niche regulator required for the maintenance of the muscle stem cell pool. EMBO Rep, e49499, doi:10.15252/embr.201949499 (2020).
10 Crane, G. M., Jeffery, E. & Morrison, S. J. Adult haematopoietic stem cell niches. Nat Rev Immunol 17, 573-590, doi:10.1038/nri.2017.53 (2017).
11 Schofield, R. The relationship between the spleen colony-forming cell and the haemopoietic stem cell. Blood Cells 4, 7-25 (1978).
12 Yin, H., Price, F. & Rudnicki, M. A. Satellite cells and the muscle stem cell niche. Physiol Rev 93, 23-67, doi:10.1152/physrev.00043.2011 (2013).
13 Wang, Y. X. et al. EGFR-Aurka Signaling Rescues Polarity and Regeneration Defects in Dystrophin-Deficient Muscle Stem Cells by Increasing Asymmetric Divisions. Cell Stem Cell 24, 419-432 e416, doi:10.1016/j.stem.2019.01.002 (2019).
14 Blackburn, D. M. et al. High-resolution genome-wide expression analysis of single myofibers using SMART-Seq. J Biol Chem 294, 20097-20108, doi:10.1074/jbc.RA119.011506 (2019).
15 Kuswanto, W. et al. Poor Repair of Skeletal Muscle in Aging Mice Reflects a Defect in Local, Interleukin-33-Dependent Accumulation of Regulatory T Cells. Immunity 44, 355-367, doi:10.1016/j.immuni.2016.01.009 (2016).
16 Lukjanenko, L. et al. Aging Disrupts Muscle Stem Cell Function by Impairing Matricellular WISP1 Secretion from Fibro-Adipogenic Progenitors. Cell Stem Cell 24, 433-446 e437, doi:10.1016/j.stem.2018.12.014 (2019).
17 Martinez, F. O. & Gordon, S. The M1 and M2 paradigm of macrophage activation: time for reassessment. F1000Prime Rep 6, 13, doi:10.12703/P6-13 (2014).
18 Tidball, J. G. & Welc, S. S. Macrophage-Derived IGF-1 Is a Potent Coordinator of Myogenesis and Inflammation in Regenerating Muscle. Mol Ther 23, 1134-1135, doi:10.1038/mt.2015.97 (2015).
19 Varga, T. et al. Macrophage PPARgamma, a Lipid Activated Transcription Factor Controls the Growth Factor GDF3 and Skeletal Muscle Regeneration. Immunity 45, 1038-1051, doi:10.1016/j.immuni.2016.10.016 (2016).
20 Lemos, D. R. et al. Nilotinib reduces muscle fibrosis in chronic muscle injury by promoting TNF-mediated apoptosis of fibro/adipogenic progenitors. Nat Med 21, 786-794, doi:10.1038/nm.3869 (2015).
21 Blackburn, D. M., Lazure, F. & Soleimani, V. D. SMART approaches for genome-wide analyses of skeletal muscle stem and niche cells. Crit Rev Biochem Mol Biol 56, 284-300, doi:10.1080/10409238.2021.1908950 (2021).
22 Shefer, G., Rauner, G., Yablonka-Reuveni, Z. & Benayahu, D. Reduced satellite cell numbers and myogenic capacity in aging can be alleviated by endurance exercise. PLoS One 5, e13307, doi:10.1371/journal.pone.0013307 (2010).
23 Wang, Y., Wehling-Henricks, M., Samengo, G. & Tidball, J. G. Increases of M2a macrophages and fibrosis in aging muscle are influenced by bone marrow aging and negatively regulated by muscle-derived nitric oxide. Aging Cell 14, 678-688, doi:10.1111/acel.12350 (2015).
24 Hamrick, M. W., McGee-Lawrence, M. E. & Frechette, D. M. Fatty Infiltration of Skeletal Muscle: Mechanisms and Comparisons with Bone Marrow Adiposity. Front Endocrinol (Lausanne) 7, 69, doi:10.3389/fendo.2016.00069 (2016).
25 Chakkalakal, J. V., Jones, K. M., Basson, M. A. & Brack, A. S. The aged niche disrupts muscle stem cell quiescence. Nature 490, 355-360, doi:10.1038/nature11438 (2012).
26 Picelli, S. et al. Full-length RNA-seq from single cells using Smart-seq2. Nat Protoc 9, 171-181, doi:10.1038/nprot.2014.006 (2014).
27 De Micheli, A. J., Spector, J. A., Elemento, O. & Cosgrove, B. D. A reference single-cell transcriptomic atlas of human skeletal muscle tissue reveals bifurcated muscle stem cell populations. Skelet Muscle 10, 19, doi:10.1186/s13395-020-00236-3 (2020).
28 Lazure, F. et al. Transcriptional Reprogramming of Skeletal Muscle Stem Cells by the Niche Environment. bioRxiv, 2021.2005.2025.445621, doi:10.1101/2021.05.25.445621 (2021).
29 Lacraz, G. et al. Increased Stiffness in Aged Skeletal Muscle Impairs Muscle Progenitor Cell Proliferative Activity. PLoS One 10, e0136217, doi:10.1371/journal.pone.0136217 (2015).
30 Longo, S. K., Guo, M. G., Ji, A. L. & Khavari, P. A. Integrating single-cell and spatial transcriptomics to elucidate intercellular tissue dynamics. Nat Rev Genet, doi:10.1038/s41576-021-00370-8 (2021).
31 Garg, K. & Boppart, M. D. Influence of exercise and aging on extracellular matrix composition in the skeletal muscle stem cell niche. J Appl Physiol (1985) 121, 1053-1058, doi:10.1152/japplphysiol.00594.2016 (2016).
32 Distefano, G. & Goodpaster, B. H. Effects of Exercise and Aging on Skeletal Muscle. Cold Spring Harb Perspect Med 8, doi:10.1101/cshperspect.a029785 (2018).
Photo Credit: Image of an isolated EDL myofiber and its associated muscle stem cells after 48 hours in culture, courtesy of Darren Blackburn.